The Exoplanetary Terrestrial Ion Chemistry model
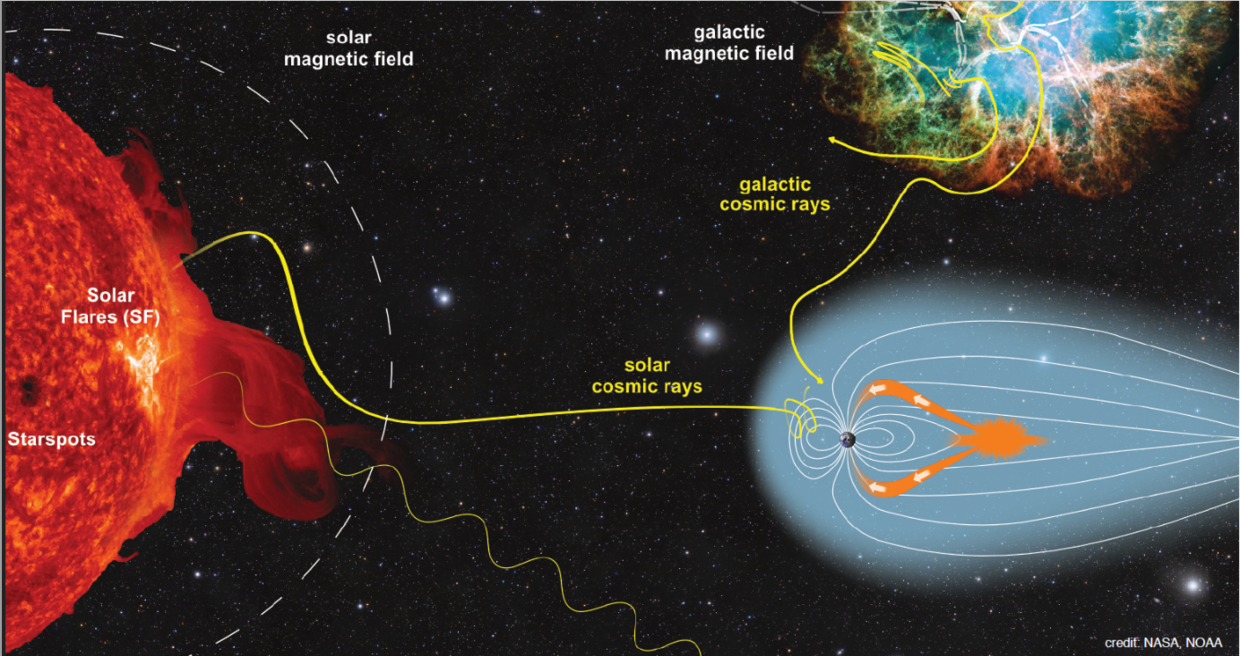
We study the influence of energetic stellar and cosmic particles on the atmospheres of Earth and exoplanets. The particle stream, consisting mainly of high-energy protons and electrons, hits the prevailing atmosphere and loses its energy through particle collisions on its way to the surface. The resulting particle showers of charged and neutral particles influence the composition of the atmosphere. These primary processes of collision, ionization and subsequent neutral and ion-chemistry are simulated by the Exoplanetary Terrestrial Ion Chemistry model ExoTIC. As a further development of the UBIC model for the Earth's atmosphere containing a simulation of top-of-atmosphere stellar radiation based on stellar spectrum and planetary orbital parameters, ExoTIC can be applied also to exoplanetary atmospheres. ExoTIC is currently used for the Earth atmosphere in the project HEPIC, for exoplanetary atmospheres in the project INCREASE.
How does ExoTIC work?
ExoTIC is a 1-dimensional stacked-box model of the atmospheric neutral and ion composition. The ion-chemistry is based on the UBIC model [Winkler et al., 2009] developed for the terrestrial middle atmosphere; the neutral chemistry is based on the SLIMCAT model [Chipperfield, 1999]. It simulates the behavior of neutral and charged trace components based on a time-varying, altitude dependent atmospheric ionization rate in a moderately charged atmosphere, and determines the time evolution of 106 charged and 58 neutral species which interact due to neutral–ion, and ion–ion gas-phase reactions, as well as photolysis and photoelectron attachment and detachment reactions but no vertical diffusion or transport [Sinnhuber et al., 2012].
The ionization is driven by prescribed ionization rates and by EUV photoionization, with the primary positive charges being distributed onto N2, N, O2 and O and balanced with electrons [Sinnhuber et al., 2012]. These rates of the primary ions are calculated by ionization cross-sections based on [Rusch et al., 1981] and [Jones and Rees, 1973]. All of the processes like dissociation and dissociative ionization of O2 and N2 as well as ionization of O2, N2 and O can form the excited states of N, O, N2+, N+ and NO+ which are also included in the model.
The solar/stellar radiation flux at the top of the atmosphere as well as an initial neutral atmosphere and the rate of atmospheric ionization can be prescribed externally. The vertical resolution and vertical range are determined by an initial atmosphere.
The neutral chemistry and the ion-chemistry are calculated iteratively as follows:
- [1] The neutral model is time dependent and calculates the volume mixing ratios of the neutral species with a variable time-step.
- [2] An equilibrium state of the ion composition is calculated due to primary ionization, positive and negative ion chemistry for the neutral atmosphere at prescribed time-intervals and altitudes, typically every hour.
- [3] The net effective production or loss rates of neutral species is calculated from the equilibrium state.
- [4] These net effective production/loss rates are then fed back to the 1-D neutral chemistry model which solves for the neutral atmospheric state transiently using these net effective production/loss reactions as well as neutral photochemistry reactions until the ion chemistry module is called again.
More details with a full list of the reactions, reactions rates and references for the reactions rates used for the positive ion-chemistry can be found in [Sinnhuber et al., 2012]. A newer version of the model is described in [Herbst et al., 2021].
References
- Chipperfield, M., Multiannual simulations with a three-dimensional
chemical transport model, JGR, 1999. - Herbst, K., Grenfell, J. L.; Sinnhuber, M.; Wunderlich, F., INCREASE: An updated model suite to study the INfluence of Cosmic Rays on Exoplanetary AtmoSpherEs, Astronomische Nachrichten. doi:10.1002/asna.20210072, 2021.
- Jones, R. and Rees, M.: Time dependent studies of the aurora—I. Ion density and composition, Planetary and Space Science, 21, 537–557, https://doi.org/https://doi.org/10.1016/0032-0633(73)90069-X, 1973.
- Rusch, D., Gérard, J.-C., Solomon, S., Crutzen, P., and Reid, G.: The effect of particle precipitation events on the neutral and ion chemistry of the middle atmosphere—I. Odd nitrogen, Planetary and Space Science, 29, 767–774, https://doi.org/https://doi.org/10.1016/0032-0633(81)90048-9, 1981.
- Sinnhuber, M., Nieder, H., and Wieters, N.: Energetic Particle Precipitation and the Chemistry of the Mesosphere/Lower Thermosphere, Surveys in Geophysics, 33, https://doi.org/10.1007/s10712-012-9201-3, 2012.
- Winkler, H., Kazeminejad, S., Sinnhuber, M., Kallenrode, M.-B., and Notholt, J.: Conversion of mesospheric HCl into active chlorine during the solar proton event in July 2000 in the northern polar region, Journal of Geophysical Research: Atmospheres, 114, https://doi.org/https://doi.org/10.1029/2008JD011587, 2009.